Titanium Dioxide (TiO2) is a material that has captured significant attention across various scientific and industrial fields. Renowned for its exceptional properties, including chemical stability, semiconductor behavior, and powerful photocatalytic activity, TiO2 serves as a foundational material for a specific class of electrodes: the Titanium Dioxide Electrode, commonly abbreviated as the TiO₂ Electrode. These electrodes, often constructed as films, powders, or coatings on conductive substrates, are pivotal in driving innovation in areas ranging from environmental remediation and energy conversion to biomedical devices.
Leveraging TiO2‘s unique interaction with light and its robust chemical nature, TiO₂ electrodes enable highly efficient electrochemical and photoelectrochemical processes, making them essential tools in modern research and technology.
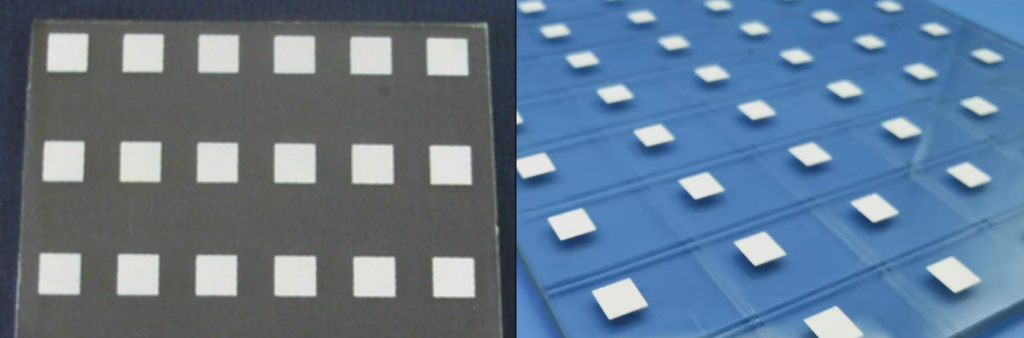
What Defines a TiO₂ Electrode?
A TiO₂ electrode is essentially an electrode system where titanium dioxide is the primary active material. While sometimes consisting of bulk TiO2, they are most frequently fabricated by applying a layer or nanostructure of TiO2 onto a conductive support material. This support, such as Indium Tin Oxide (ITO) glass, Fluorine-doped Tin Oxide (FTO) glass, or various carbon materials, provides the necessary electrical conductivity, as pure TiO2 itself is a semiconductor with relatively low inherent conductivity in the dark.
The key to the TiO₂ electrode’s function lies in the properties of titanium dioxide: its wide band gap semiconductor nature allows it to absorb UV light (and visible light if modified), generating electron-hole pairs that can drive redox reactions at the electrode surface.
Fabrication and Preparation Processes
The performance of a TiO₂ electrode is highly dependent on its morphology (nanoparticles, nanotubes, thin films) and crystalline structure. Common preparation methods include:
- Paste Preparation: High-purity TiO2 powder (often in the anatase or rutile phase, with anatase favored for photocatalysis) is mixed with binders (like organic polymers) and solvents (often including dilute acids like nitric acid) to form a viscous, homogeneous paste.
- Coating on Conductive Substrate: The prepared paste is applied onto a pre-cleaned conductive substrate. Techniques like doctor blade coating (for precise thickness), dip coating, screen printing, or spray coating are used to create a uniform TiO2 layer.
- Sintering: The coated substrate is heated in a furnace to temperatures typically ranging from 400°C to 500°C. This critical step burns off organic binders, crystallizes the TiO2 particles (improving inter-particle connectivity), enhances adhesion to the substrate, and forms a stable, porous film structure crucial for diffusion and charge transport.
- Nanostructuring (Advanced Methods): To maximize surface area and tailor properties, advanced techniques create specific nanostructures:
- Electrochemical Anodization: Used to grow highly ordered TiO2 nanotube arrays directly on a titanium metal substrate.
- Hydrothermal Synthesis: Can produce various TiO2 nanostructures in solution.
- Electrodeposition: Allows for one-step formation of crystalline TiO2 films with controlled morphology. These nanostructuring methods dramatically increase the electrode’s active surface area, providing more reaction sites and improving charge carrier separation and transport, which is vital for enhancing photocatalytic and electrochemical performance.
- Electrochemical Anodization: Used to grow highly ordered TiO2 nanotube arrays directly on a titanium metal substrate.
Key Properties and Advantages
TiO₂ electrodes possess a suite of properties that make them highly advantageous in diverse applications:
- High Chemical Stability: TiO2 is remarkably inert and stable under harsh chemical conditions, including strong acids, bases, and highly oxidative environments. This prevents electrode degradation and contamination of the reaction medium.
- Exceptional Photocatalytic Activity: This is a standout feature. Under UV light irradiation (or visible light if sensitized or doped), TiO2 absorbs photons with energy greater than its band gap, generating electron-hole (e−/h+) pairs. These highly reactive charge carriers migrate to the surface and initiate redox reactions, driving the degradation of organic pollutants or the splitting of water.
- Biocompatibility: TiO2 is non-toxic and biocompatible, making it suitable for direct contact with biological systems in medical implants, biosensors, and drug delivery applications. It can even exhibit antibacterial properties.
- Large Surface Area and Porosity: Especially when prepared using nanostructuring techniques, TiO₂ films offer a very high effective surface area. This porosity facilitates electrolyte penetration and provides numerous active sites for surface reactions, greatly enhancing catalytic efficiency and charge transfer.
- Cost-Effectiveness and Abundance: Titanium dioxide is one of the most abundant metal oxides on Earth and is relatively inexpensive to produce compared to electrodes based on noble metals like platinum or iridium.
- Versatility: The combination of stability, photoactivity, and the ability to modify its structure and combine it with various substrates allows TiO₂ electrodes to be adapted for a wide spectrum of applications.
Role in Electrochemical and Photoelectrochemical Systems
TiO₂ electrodes function primarily by mediating electron transfer processes, often initiated by light:
- Photoelectrochemical Reactions: When illuminated with UV light, TiO2 generates e−/h+ pairs. Electrons can be transferred to the conductive substrate and external circuit, while holes migrate to the surface to oxidize adsorbed species (e.g., water, pollutants). This forms the basis for photocatalytic degradation and water splitting (the Honda-Fujishima effect).
- Electrocatalysis: TiO₂ can directly participate as a catalyst or, more commonly, serve as a highly stable and porous support material for depositing other catalysts (like metal nanoparticles) to facilitate various redox reactions.
- Charge Separation and Transport: The morphology, particularly nanostructures like nanotubes or porous films, is engineered to optimize the separation of photogenerated e−/h+ pairs and provide efficient pathways for charge carriers to reach the electrode surface or the external circuit, minimizing wasteful recombination.
- Surface Adsorption: The surface chemistry of TiO2 allows for the adsorption of molecules and ions from the electrolyte, positioning them for subsequent photocatalytic or electrochemical reactions.
Diverse Usage Scenarios
TiO₂ electrodes are implemented in a variety of practical and cutting-edge scenarios:
- Wastewater Treatment Plants: Used in advanced oxidation processes (AOPs) for the photocatalytic degradation of recalcitrant organic contaminants and pathogens, enhancing water purification.
- Solar Hydrogen Production Facilities: Serve as photoanodes in photoelectrochemical cells to split water using sunlight (or UV light), contributing to clean hydrogen fuel generation.
- Energy Storage Devices: Employed as anode materials in next-generation Lithium-ion and Sodium-ion batteries due to their good capacity, rate capability, and safety profile. Also used in supercapacitors and as electron transport layers in Dye-Sensitized Solar Cells (DSSCs).
- Biomedical Implants and Sensors: Integrated into biosensors as stable platforms for detecting biological molecules and used in orthopedic implants for their biocompatibility and ability to promote bone growth. Research explores their potential in photodynamic therapy for cancer.
- Air Purification Systems: Used as photocatalytic filters or coatings to decompose Volatile Organic Compounds (VOCs) and nitrogen oxides (NOx) in polluted air streams.
- Coatings for Self-Cleaning and Anti-Fogging Surfaces: Utilized on glass, tiles, or building materials. Under UV light, they become superhydrophilic (self-cleaning with water) or exhibit photocatalytic degradation of dirt.
Summary Table
Aspect | Details |
Main Applications | Photocatalysis (env. remediation), Water Splitting, Energy Storage/Conversion, Biomedical, Protective Coatings |
Preparation | Paste formulation, Coating on conductive substrate (ITO, carbon), Sintering, Nanostructuring (Anodization, Hydrothermal) |
Key Advantages | High Chemical Stability, Photocatalytic Activity (UV light), Biocompatibility, Large Surface Area/Porosity, Cost-Effective, Versatility |
Electrochemical Role | Drives redox reactions via photogenerated charge carriers, Electrocatalyst/Support, Charge Transport, Adsorption |
Typical Usage Scenarios | Wastewater Treatment, Hydrogen Production, Batteries, Medical Implants/Sensors, Air Purification, Self-Cleaning Surfaces |
This comprehensive overview highlights the multifaceted nature and broad applicability of Titanium Dioxide Electrodes, underscoring their importance in current and future technological advancements.
Partnering with an Expert Supplier
Developing and producing high-performance Titanium Dioxide Electrodes requires specialized knowledge in materials science, nanotechnology, and electrode fabrication. A leading manufacturer and supplier specializing in titanium and titanium oxide electrodes brings the necessary expertise, advanced processing capabilities, and rigorous quality control to produce TiO₂ electrodes with precisely controlled morphology, crystalline phase, surface area, and electronic properties. Look for a partner capable of providing customized production – tailoring the substrate, TiO2 form (nanoparticles, nanotubes, films), coating method, and potentially doping to meet the specific requirements of your research, product development, or industrial application. Such a collaboration is crucial for leveraging the full potential of TiO₂ electrodes.
Titanium Dioxide (TiO₂) electrodes are highly versatile and stable materials playing an increasingly vital role in addressing global challenges related to environmental protection, sustainable energy, and healthcare. Their unique photocatalytic, electrochemical, and biocompatible properties, combined with their cost-effectiveness and abundance, position them at the forefront of research and industrial implementation. As preparation techniques become more refined, the applications and performance of TiO₂ electrodes will undoubtedly continue to expand, promising innovative solutions for a sustainable future.
FAQs About Titanium Dioxide (TiO₂) Electrodes
What are the main uses of TiO₂ electrodes?
TiO₂ electrodes are primarily used in photocatalysis (for breaking down pollutants), photoelectrochemical water splitting (for hydrogen production), energy storage and conversion devices (like batteries and solar cells), and biomedical applications (biosensors, implants).
How are TiO₂ electrodes typically made?
A common method involves preparing a TiO2 paste, coating it onto a conductive substrate (like ITO glass or carbon), and then sintering it at high temperatures to form a stable, porous film. Advanced methods like electrochemical anodization are used to create nanostructures such as nanotube arrays.
What are the advantages of using TiO₂ electrodes compared to other materials?
Key advantages include their high chemical stability in harsh conditions, unique photocatalytic activity under UV light, biocompatibility for medical uses, large controllable surface area (especially when nanostructured), cost-effectiveness and abundance compared to noble metals, and versatility across many applications.
What is the role of TiO₂ electrodes in electrochemical reactions?
In photoelectrochemical reactions, they generate electron-hole pairs under light to drive redox reactions (like water splitting or pollutant degradation). They can also act as electrocatalysts or catalyst supports, facilitate charge separation and transport, and enable surface adsorption of reactants.
Are TiO₂ electrodes used as anodes or cathodes?
TiO₂ can function as an anode or cathode depending on the specific reaction and applied potential. In photoelectrochemical water splitting, they are typically used as photoanodes (where oxidation occurs). In batteries, they can serve as anode materials (where lithium or sodium ions are inserted/extracted). Their role depends on the specific application and electrochemical conditions.